SCIENTIFIC COMMUNIQUÉ XI: Developing Novel Treatments for Arterial Ischaemic Stroke Patients
Abstract
One in four people globally will suffer from a stroke in their lifetime. For those who survive, the consequences are often long-lasting, with many suffering from permanent disabilities that result in symptoms, including cognitive deterioration and movement impairments.
Strokes are classed as either haemorrhagic or ischaemic, with the latter responsible for over 85% of all stroke cases. Presently, there are only two recognised therapies for an ischaemic stroke, as recommended by the 2018 American Stroke Association treatment guidelines: thrombolysis and endovascular thrombectomy. However, due to the extremely narrow therapeutic window of each treatment, only a few stroke patients actually receive these interventions. Administration must occur within a maximum of 4.5 and 6 hours (for thrombolysis and thrombectomy, respectively) following stroke onset. Outside of this time frame, these treatments are no longer considered safe or effective, as they would instead place the patient at a high risk for secondary complications such as brain bleeding, which only further contributes to poor prognosis.
According to the Australian Stroke Association, only 36% of stroke patients arrive at an emergency room within 4.5 hours. Considering the time then required to diagnose the condition, and possibly the necessity to transfer patients to specialised stroke units, the percentage of eligible patients drops dramatically, with only 13% receiving this most vital medical care.
A stroke can occur at anytime and anywhere. Even the most minor delays can be the difference between receiving treatment or not. The annual occurrence of stroke is surging, with stroke risk factors such as hypertension and diabetes remaining an evergrowing issue worldwide. The need for new and viable interventions, which are suitable for a wider stroke population than currently available, is critical.
A multitude of independent studies have examined the therapeutic potential of melanocortins in improving ischaemic stroke outcomes. Collectively, their preclinical results indicate the favourable use of these hormones, and their analogues, in this prevalent disease. Although this suggests the prospective role of melanocortins in an ischaemic stroke, further in-human clinical trials are mandatory to confirm this hypothesis.
Introduction
The human body is comprised of many different tissues that each demand a continuous, and ample supply of essential nutrients (such as glucose, ions, and oxygen) to produce energy. It is then this energy which fuels and sustains normal organ survival.
The body’s blood vessels (specifically its arterial system), exist to transport large volumes of nutrient enrich blood directly from the heart to these targets. It must be emphasised that the energy expenditure differs considerably between each individual organ. This means the amount of blood needed to sustain each organ will vary, dependent on it’s metabolic needs.
The brain, for example, is one of the most nutrient consuming organs in the body – even at rest. To help coordinate its normal everyday tasks, human physiology has evolved to contain its own network of arteries that exclusively irrigate the brain. This is known as the cerebral arterial system (see SCIENTIFIC COMMUNIQUÉ VII).
The three largest arteries, known as the paired cerebral arteries (the anterior cerebral artery (ACA), the middle cerebral artery (MCA) and the posterior cerebral artery (PCA)) arise from the main vessels of the heart and comprise the cerebral arterial system. These arteries each ascend into the depths and crevasses of the brain, ensuring all regions of this organ receive a plentiful supply of blood, and thereby nutrients.
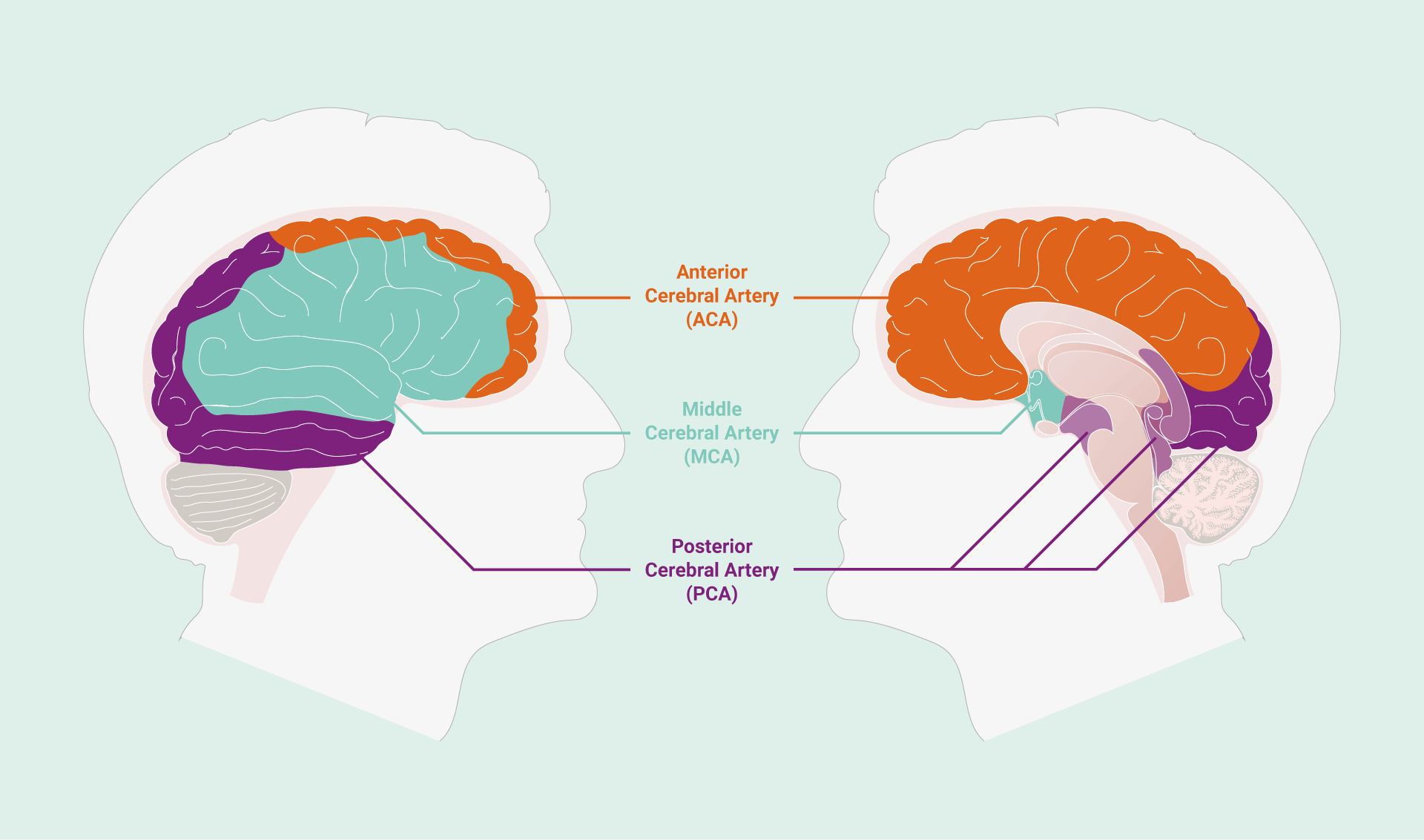
The middle cerebral artery (MCA) supplies oxygenated blood to the lateral surfaces of the brain’s left and right hemispheres, as well as medial structures such as the basal ganglia and internal capsule.
Yet, when this blood supply is disrupted, a severe and life-threatening disease rapidly develops as nutrients are prevented from reaching the brain’s respiring tissues. This disease is known as a stroke. Defined as an acute interruption of blood flow to the brain, strokes are one of the leading causes of permanent disability and mortality. It is estimated that roughly 15 million people worldwide suffer from a stroke every year, with one person dying from a stroke every four minutes in the US.
In this communiqué, we will breakdown the key aspects of a stroke, examine current treatments, and describe potential alternative therapeutics that may be applicable in the treatment of strokes.
Ischaemic Strokes and the Middle Cerebral Artery
Distinguished by its cause, or aetiology, strokes can be defined as either haemorrhagic or ischaemic.
Accounting for more than 85% of all stroke cases, the latter arises from a physical obstruction inhibiting the normal movement of blood. A haemorrhagic stroke appears following the bursting of a blood vessel directly within the brain. An ischaemic stroke may also be referred to as an arterial ischaemic stroke (AIS) if the blockage lies within one of the brain’s arteries – which is the case for almost all ischaemic strokes.
The MCA is the largest of the cerebral arteries and is also a direct descendant of the large internal carotid artery (see SCIENTIFIC COMMUNIQUÉ VII). However, due to its size and prime location, the MCA is one of the most common sites for debris to become lodged. In fact, over 50% of AIS cases occur due to the presence of a blockage within the MCA.
Broken down into four distinct segments, the MCA has an organisation that can be compared to the structure of a tree. This is because the M1, or first segment of the MCA, divides into two smaller segments, known as the M2 arteries. Due to bifurcation, the M2 arteries are much narrower in size. A similar pattern follows for the M3 and M4 arteries, each a division of the previous MCA segment. As expected, the M4 segment is comprised of the narrowest and most distal arteries. In this analogue, the M1 exists as the trunk of the tree, while the M4 represents the outermost twigs. Collectively, these blood vessels supply almost the entire medial and lateral surfaces of the brain’s left and right hemispheres (Figure 1).
When an obstruction occurs in the MCA, oxygen is blocked off to vast and extensive areas of the brain, which all become vulnerable to tissue death. Regions such as the motor-sensory cortex and visual fields are perfused by the MCA, which explains why during an MCA stroke, patients will experience an inability to move their face and legs, as well as blurred sight.
The most threatening type of AIS occurs when an impasse is found within the first segment, or M1, of the MCA. This is because not only is the M1 responsible for supplying the medial structures of the brain with blood (Figure 1), it is also liable for supplying blood to the extremital segments of the MCA (M2 to M4). This means each region of the brain perfused by the M2 to M4 arteries will suffer from oxygen deprivation as well. Without intervention, an M1 obstruction will most often cause the largest volumetric destruction of brain tissue.
15 million people worldwide suffer from a stroke every year, with one person dying from a stroke every four minutes in the US.
The Pathology of an Arterial Ischaemic Stroke
The pathology of an AIS is sequential and can crudely be broken down into two distinct phases of damage.
Phase 1: The Ischaemic Cascade
The majority of all ischaemic strokes first begin from complications of the vascular disease atherosclerosis (see SCIENTIFIC COMMUNIQUÉ VII). During atherosclerosis, hardened plaques develop in the wall of a diseased artery. Their presence stiffens and narrows the vessel, which not only causes blood pressure to rise (hypertension) but starts to restrict normal blood flow to targeted tissues. At this stage the condition isn’t considered life-threatening, but when in more advanced states of development, these plaques are very unstable. This means they are prone to rupturing under stressful conditions, one of which includes prolonged hypertension. Upon rupturing, the contents of the plaque spills messily across the lumen of the artery. The body interprets this fissuring event as an injury and, in response, the affected blood vessel constricts suddenly. Blood coagulation is then initiated in an attempt to temporarily thwart the damage, yet this only contributes to the formation of an impending blood clot within the artery.
Clots can manifest either indirectly within the brain’s arteries (thrombi) or alternatively within a peripheral blood vessel where the clot is then transported towards the head and becomes lodged in a cerebral artery (emboli). In either setting, inadequate supply of blood to the brain starts to induce hypoxic (lack of oxygen) and hypoglycaemic (low sugar, or glucose) conditions, as the brain tissue is starved of these crucial compounds. The neurones and associated cells that make up the brain are immediately affected. Oxygen is needed by all the cells of the body to make energy through a process known as aerobic respiration. Without enough oxygen, brain cells have to strategically conserve the small amount of energy they have left by switching to ‘cell survival mode’, or anaerobic respiration. While some energy is made by the cell through this alternative or residual process, it is only able to harvest a minimal amount of energy (18x≈less energy than aerobic respiration). For most cells, this means only performing tasks that are critical to keep the cell alive.
To make matters worse, this is only a temporary fix. Eventually the neurones and associated brain cells will be drained of their resources if the obstruction remains, and with this comes inevitable cell death through a process known as the ischaemic cascade. Within one minute of oxygen deprivation, over 1.9 million neurones are irreversibly lost through this process. With the average brain comprised of 86 billion neurones, this is an eye-watering amount.
The ischaemic cascade occurs within seconds to minutes, however, there is a second phase of damage that occurs following AIS onset. This is known as the breakdown of the blood brain barrier (BBB).
Within one minute of oxygen deprivation, over 1.9 million neurones are irreversibly lost.
Phase 2: The Blood Brain Barrier Breakdown
As previously discussed, the main purpose of our arterial system is to deliver nutrients to our organs. Arteries, while useful at carrying and distributing large volumes of high pressured blood, are not structurally adapted to directly allow for gas and nutrient exchange. This is due to the circumferential layer of vascular smooth muscle and elastic fibres that comprise the walls of the arteries, creating significant resistance to the diffusion process. The capillaries are found downstream to the body’s arteries and are a key component of the arterial system. The walls of these capillaries are typically very thin – usually a single cell thick. It is this adaptation that allows for the rapid exchange of substances from the blood, across into the tissue (and vice versa). Without capillaries, this exchange would be inhibited, and the body’s tissues would eventually undergo death.
The cerebral arterial system is also composed of a web of capillaries, but unlike the peripheral circulatory system, the microvasculature in the central nervous system is highly specialised. Though a sole layer of endothelial cells still lines the inner walls of the cerebral capillaries, these cells are packed much closer together, creating an almost impermeable barrier. This interface is known as the BBB. A sheath of additional cells also coats the outer layer of the capillaries. Comprised of astrocytes and pericytes cells, as well as the brain’s extracellular matrix, researchers have labelled this supplementary arrangement the neurovascular unit (NVU) (Figure 2).
Though the presence of the neurovascular unit may seem counterintuitive to the function of the body’s capillaries, the superimposition of these additional cells, firmly interconnected alongside the BBB, prevents unwanted substances, such as toxins and pathogenic material, from entering the brain and causing disease. It also regulates the trafficking of substances such as fluid and solutes in and out of the brain, to ensure an optimum microenvironment is maintained within the brain tissue at all times.
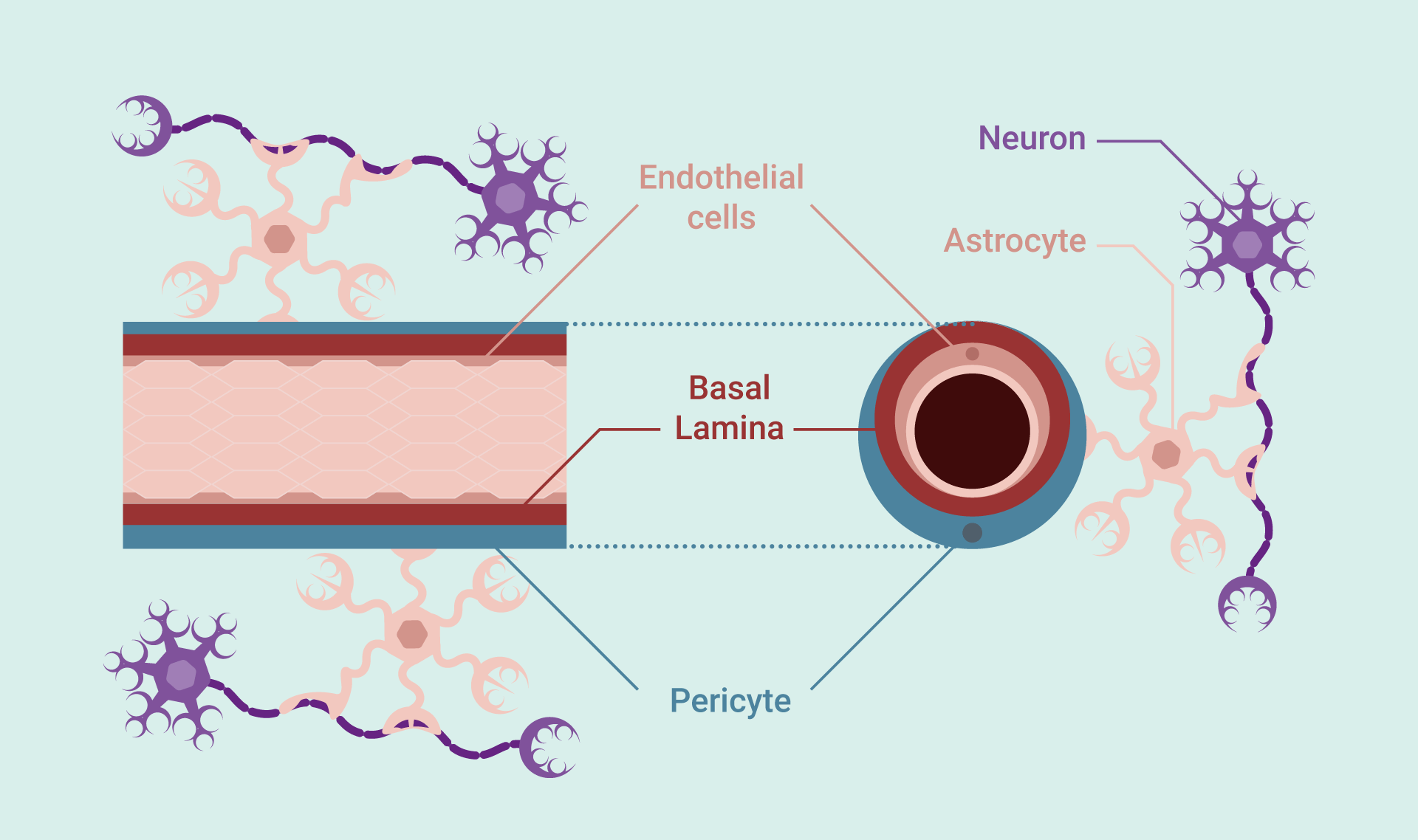
The inner wall of the brain’s capillaries is comprised of a single layer of endothelial cells, tightly adjoined to one another by multicomplex proteins, known as tight junctions. The presence of these tight junctions reduces the permeability of the capillaries and restricts the movement of unwanted substances, bi-directionally. The presence of cell membrane transporters helps enable the movement of vital molecules across endothelium.
A basal lamina encases the endothelium, to which pericyte cells are connected to. The pericytes help maintain the blood brain barrier (BBB) by regulating the trafficking of cells and substances. Astrocytes and their end-feet help associate the BBB to nearby neurones. Overall, the NVU is responsible for maintaining the highly selective BBB and ensuring cerebral homeostasis.
One of the main causes of BBB breakdown during AIS is the presence of immune cells (known as microglia). Following necrotic and apoptotic cell death in the ischaemic core, the brain’s resident microglia are activated within the first few hours of disease progression. These cells are phagocytotic, meaning they operate to engulf dead cells and other cellular debris, facilitating ischaemic waste clearance. There are, however, only a small number of microglial naturally present within the brain tissue. To aid this process, they release signalling molecules, known as cytokines, which attract a surplus of immune cells from the circulating blood. This action initiates neuroinflammation.
As these peripheral immune cells migrate across the BBB into the brain tissue, they excel BBB injury by releasing enzymes, such as the metalloproteinases, which cleave away at the barrier. It is this action that is the main cause of BBB breakdown, however, disruption to the barrier can also be influenced by other factors.
As expected, when the BBB is structurally disturbed in this fashion, it distorts the BBB’s defensive function. Strict selective permeability is lost, which means substances from the blood can now leak into the brain tissue. This includes fluid, which pools into the brain (vasogenic oedema). Following extensive breakdown, blood may also seep out of the vessels and cause haemorrhaging. Together, this causes the brain to swell and intracranial pressure rises, placing further stress upon the already vulnerable cerebral tissue. Clinical symptoms such as lost vision, confusion, and vomiting may be seen. Once this has started to occur, prognosis is extremely poor, although these events typically occur several hours to days after AIS initiation.
Saving the Penumbra
In the brain of an individual experiencing an AIS, there are two main areas of injury that can be identified: the necrotic core and the penumbra. The former describes the region of brain tissue that was directly perfused by the occluded artery’s downstream capillaries, and rapidly victimised by the ischaemic cascade within minutes.
The necrotic core is simply a cluster of irreversibly dead brain cells. Even if oxygen was to be reinstated to this area, the cells would not revive, nor is regeneration possible. It must be explained that the necrotic core is not a static entity but is in fact dynamic. As brain tissue continues to experience oxygen deprivation, and BBB breakdown is instigated, the number of brain cells which succumb to death increases – this seen as the core expanding further outwards, engulfing broader areas of the brain. Clinically advanced strokes exhibit an extensive necrotic core, associated with poor prognosis.
The penumbra describes viable, and therefore, salvageable cerebral tissue that can retain its functionality for more than several hours after the ischaemic event. The prolonged survival of penumbra tissue is credited to the brain’s collateral artery circulation. While the primary vessel conduit fails, these subsidiary channels act as a bypass for circulating blood. These vessels continue to supply this area with a reduced volume of blood – enough to sustain cell survival temporarily. Darby et al, however, stated the volume of reversibly damaged tissue in the penumbra decreases to just 50%, 24 hours after ischaemia. Without rapid intervention, the penumbra is destined to follow the same path as the cells in the necrotic core.
The phrase ‘time is brain’ is often heard in neurology, referring to the progressive loss of functional brain tissue, as the time taken to achieve reperfusion delays. Rather, ‘time is penumbra’ is probably a more accurate depiction of this definition, as the focus of all therapies for an AIS involves achieving tissue reperfusion to rescue the penumbra.
…the focus of all therapies for AIS involves achieving tissue reperfusion to rescue the penumbra.
The Current Landscape of Arterial Ischaemic Stroke Treatments
In 1996, the US Food and Drug Administration (FDA) approved the first major treatment for AIS. Known as thrombolytics, or recombinant tissue plasminogen activators (rtPA), this new class of drug was a first of its kind, and evoked high hopes for its use in improving prognostic outcomes in stroke patients.
Alteplase is currently the only FDA approved rtPA for use in stroke patients. While other thrombolytics have been successfully developed, market approval for these pharmaceuticals remains only for use in myocardial infarctions (heart attacks). Following intravenous infusion, rtPA functions by stimulating specific proteins found natively within our blood. These proteins activate a secondary protein known as plasmin, which has the potent ability to disintegrate the fibres that coat and comprise a blood clot. This action is known as fibrogenesis, as the fibrin fibres of the clot are rapidly severed away. As a result, blood perfusion to the brain is reinstated, as plasmin acts to remove this life-threatening obstruction from the cerebral arteries.
While treatment with rtPA has shown to improve patient outcomes by 1.9 times, it is not without its risks. A significant danger of thrombolytic therapy is the provocation of secondary complications which includes brain bleeding. In medical terms this is known as haemorrhagic transformation (HT) of a stroke. HT correlates to worsened survival and recovery rates as brain injury is heightened (as stated above).
As an AIS progresses, and BBB breakdown is seen, it is believed the fibrinolytic activity of rtPA actually hastens this second phase of AIS damage and increases BBB permeability. This action would also enable the accumulation of rtPA within the brain as the autoregulatory capacity of the BBB transpires. rtPA has also been noted as a potent pro-inflammatory signalling molecule. At present, we know rtPA promotes brain inflammation through the upregulation of the expression of immune related proteins, which enhances BBB failure further, leading to excessive brain bleeding.
The medical community does recognise that, during the very early stages of an AIS, rtPA’s negative actions are outweighed by its positive impact in reinstating oxygenated blood flow to the penumbra. With this in mind, the majority of current healthcare guidelines enforce a maximum 4.5 hour treatment window for thrombolytics in ischaemic strokes to promote stroke recover whilst preventing the probability of HT.
It is because of this restriction that clinics see such low therapy uptake. One study reported, on average, only 1.8 to 2.1 % of all ischaemic stroke patients received rtPA therapy. However, this unforeseen setback in managing and controlling AIS outcomes did instigate the development of a secondary AIS therapy, endovascular thrombectomy. Unlike rtPA, this newer intervention is not a drug, but instead a device. Tiny, thin flexible tubes (known as microcatheters) are inserted either through the groin or arm into the patient’s artery. This is an intricate procedure that requires the use of X-rays to help guide the surgeon through the extensive arterial network – eventually reaching the occluded artery in the brain. Upon reaching the clot, a mesh net known as a stent is then released from the tube, seizing the clot in the process. At this stage the clot is typically sucked back into the microcatheter, where the tube is then removed from the body.
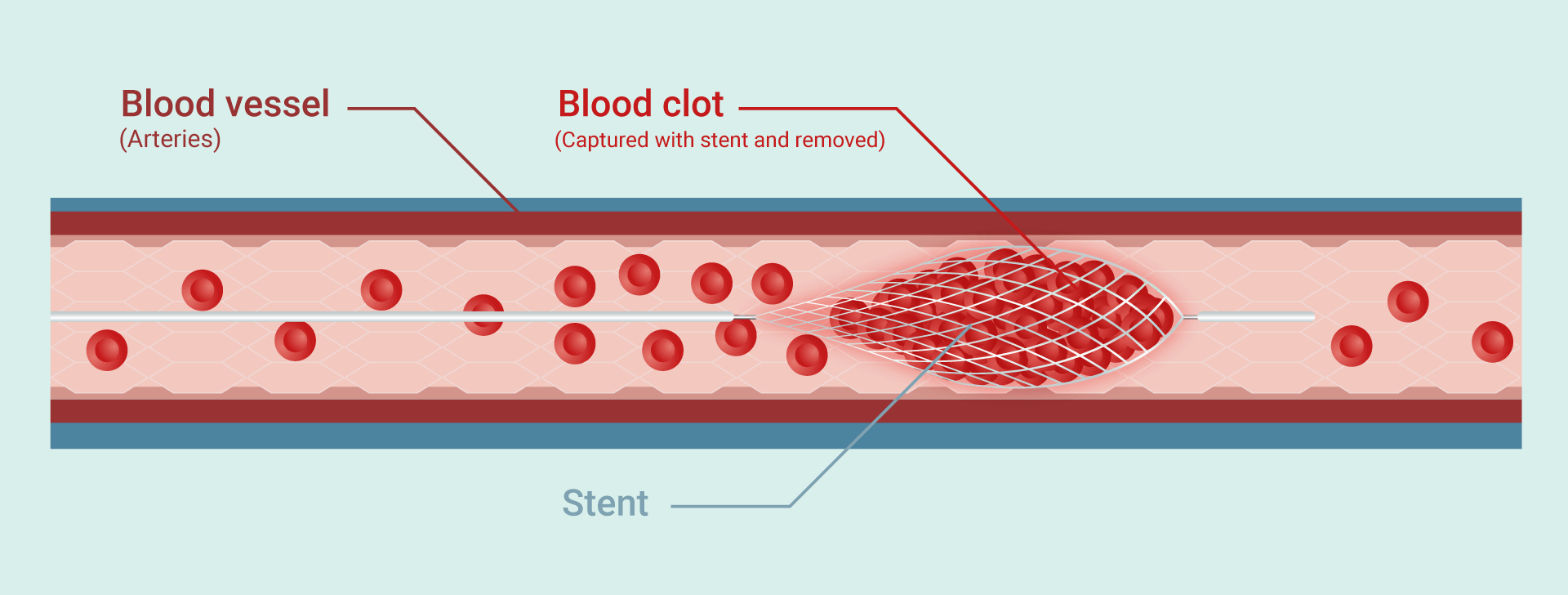
Removing a clot in this mechanical way does have its own issues. For one, the tube must be carefully guided all throughout the labyrinth that is the peripheral blood vessels, until reaching the brain. This is an extremely long journey, which is made further challenging by the loops and curvature of blood vessels. During a stroke, the affected blood vessels are tightly constricted, causing them to become extremely thin. At any point, the tube could accidently pierce the wall of an artery – leading to internal bleeding. For this reason, endovascular thrombectomy of an AIS that has occurred in the smaller arteries, such as the M2 to M4 branches of the MCA, is prohibited. Though minor, there is also a chance the clot may be inadvertently pushed deeper into the brain which will complicate treatment and further delay the time to reperfusion. The American Stroke Association guidelines suggest endovascular thrombectomy must occur within 6 hours of symptom onset. While this is slight improvement when compared to the timeframe of rtPA, it is still limiting and provides no other alternative for those patients with a blockage in one of the brain’s smaller vessels.
rtPA and thrombectomy remain the most powerful tools in improving AIS outcomes. This is a worrying statement when it is known more than 85% of the European population who suffer from an AIS don’t receive either treatment due to ineligibility.
Just over 30% of ischaemic stroke patients reach the hospital within the ‘golden hours’, arriving between one to three hours following stroke onset. While this may appear somewhat promising, this percentage is slashed to roughly 12 to 13% of patients actually receiving treatment. This is because treatment may only begin after a conclusive diagnosis of the disease can be given. Although physicians work rapidly to ensure diagnosis is completed within 60 minutes, timely questionnaires, CT, and possible MRI scans must be first given to the patients before any intervention can start. This is a critical step that cannot be excluded from the stroke process, as an incorrect diagnosis, such as identifying the stroke as haemorrhagic instead of ischaemic, would most likely lead to certain death as treatments are disease-specific.
There have been attempts to try and minimise the time taken to achieve treatment. This includes governmental run education campaigns on when to seek medical help (i.e. F.A.S.T campaign) and the introduction of mobile stroke units in some countries. The main hinderance of treatment access, however, still remains the extensive exclusion criteria that both the current standard treatments present.
Just over 30% of ischaemic stroke patients reach the hospital within the ‘golden hours… this percentage is slashed to roughly 12 to 13% of patients actually receiving treatment.
Missing Pieces of the Puzzle in Arterial Ischaemic Stroke
It is undeniably clear that the landscape for AIS intervention is incomplete. While the current treatments are successful to an extent, they discriminate against a large portion of the stroke patient population. With AIS being one of the most common causes of mortality and disability worldwide, it is fundamental that the medical industry must now propose alternative and/or additional therapies, which target AIS patients that fall outside current treatment criteria.
The melanocortins are multifunctional hormones that act upon a number of organs and tissues found in the human body, including our vascular system and brain. Numerous research groups have investigated the potential therapeutic application of melanocortins such as alpha-melanocyte-stimulating hormone (α-MSH), and their analogues, in models of AIS. In SCIENTIFIC COMMUNIQUÉ V we discussed one apparent role of α-MSH in stimulating vasodilation. As mentioned, during stroke, our disease vessels are in a continuous state of constriction. In this configuration, the narrowing of an already blocked artery further prohibits oxygenated blood from reaching the injured brain tissue, excelling poor prognosis. It is thought that, α-MSH may subsequently aid recanalisation of the affected artery through this method.
In 2016, Mykicki et al and her team demonstrated Nle4-d-Phe7-α-melanocyte-stimulating hormone (NDP-MSH), a synthetic analogue of α-MSH, exerted long-lasting neuroprotective effects in mice through reducing the effect of the ischaemic cascade that otherwise leads to accelerated neuronal cell death. Similar findings had already been found by an Italian research group led by Giuliani et al in 2006. Her team illustrated, following the induction of global cerebral ischaemia in gerbils (blocking all arteries that facilitate blood flow to the brain), when then treated with NDP-MSH, the animals showed reduced brain tissue injury, which was dose dependent. Even after administering treatment nine hours after symptom onset, the gerbils still exhibited a functional improvement. This therefore infers to the function of melanocortins in reducing the progression of the ischaemic cascade into the penumbra.
The drug was proven to have modulated and reduced the neuroinflammation response, reducing immune cell infiltration across the BBB and therefore decreasing BBB breakdown. Through BBB protection, α-MSH consequently minimised brain swelling, intracranial pressure and HT of AIS, as fluid and blood were kept enclosed within the blood vessels.
There is thus experimental evidence that suggests the melanocortins could improve neurological outcomes in patients experiencing an AIS. Although additional evidence is required, the outcome of Giuliani et al’s research was suggestive of a longer treatment time window, with similar results demonstrated in a later study by the same team. Furthermore, dissimilar to endovascular thrombectomy, this treatment is not limited to larger vessel occlusions, which overcomes the major issues associated with current therapies.
Conclusion
With 15 million people worldwide suffering from a stroke every year, it is astounding that only 13 to 15% of stroke patients are eligible to receive lifesaving treatment. More inclusive therapeutic options for AIS not only need to be developed but are vital if society wishes to see any improvements in current stroke outcomes.
Melanocortins and their analogues have shown therapeutic potential in AIS experimental studies. The abundance of evidence gathered to date is promising, however the application of melanocortins outside of in vitro (cell studies) and animal models have been non-existent thus far. The next stage of research would require the examination of these hormones in humans.
References
2015 AHA/ASA Focused Update of the 2013 Guidelines for the Early Management of Patients with Acute Ischemic Stroke Regarding Endovascular Treatment. Powers WJ, Derdeyn CP, Biller J, et al. Stroke 2015;46):3020-3035.
Adeoye, O., Hornung, R., Khatri, P., & Kleindorfer, D. (2011). Recombinant tissue-type plasminogen activator use for ischemic stroke in the United States: a doubling of treatment rates over the course of 5 years. Stroke, 42(7), 1952–1955.
American Heart Association. “Stroke Patients Who Reach Hospitals Within ‘Golden Hour’ Twice As Likely To Get Clot-busting Drug.” ScienceDaily. ScienceDaily, 28 February 2009.
Cipolla MJ. The Cerebral Circulation. San Rafael (CA): Morgan & Claypool Life Sciences; 2009. Chapter 2, Anatomy and Ultrastructure.
Demaerschalk, B. M., Kleindorfer, D. O., Adeoye, O. M., Demchuk, A. M., Fugate, J. E., Grotta, J. C., Khalessi, A. A., Levy, E. I., Palesch, Y. Y., Prabhakaran, S., Saposnik, G., Saver, J. L., Smith, E. E., & American Heart Association Stroke Council and Council on Epidemiology and Prevention (2016). Scientific Rationale for the Inclusion and Exclusion Criteria for Intravenous Alteplase in Acute Ischemic Stroke: A Statement for Healthcare Professionals From the American Heart Association/American Stroke Association. Stroke, 47(2), 581–641.
Giuliani D, Mioni C, Altavilla D, Leone S, Bazzani C, Minutoli L, Bitto A, Cainazzo MM, Marini H, Zaffe D, Botticelli AR, Pizzala R, Savio M, Necchi D, Schiöth HB, Bertolini A, Squadrito F, Guarini S Endocrinology. 2006 Mar; 147(3):1126-35.
Giuliani D, Ottani A, Mioni C, Bazzani C, Galantucci M, Minutoli L, Bitto A, Zaffe D, Botticelli AR, Squadrito F, Guarini S Eur J Pharmacol. 2007 Sep 10; 570(1-3):57-65.
Jiang X, Andjelkovic AV, Zhu L, Yang T, Bennett MVL, Chen J, Keep RF, Shi Y. Blood-brain barrier dysfunction and recovery after ischemic stroke. Prog Neurobiol. 2018 Apr-May;163-164:144-171.
Mykicki, N., Herrmann, A. M., Schwab, N., Deenen, R., Sparwasser, T., Limmer, A., Wachsmuth, L., Klotz, L., Köhrer, K., Faber, C., Wiendl, H., Luger, T. A., Meuth, S. G., & Loser, K. (2016). Melanocortin-1 receptor activation is neuroprotective in mouse models of neuroinflammatory disease. Science translational medicine, 8(362), 362ra146.
Scientific Communiqué Library
The Scientific Communiqués provide an in-depth look at the work we conduct and the rationale for developing melanocortins.
- Engine, Ignition, and Fuel: Light and Skin Interaction
- Ligand Binding and the Melanocortin-1 Receptor (MC1R)
- Afamelanotide and the Human Genome
- MC1R Allelic Variants and Skin Cancer Risk
- Regulating the Vascular System: Nitric Oxide
- Ultraviolet Radiation Damage and Oxidative Stress in Skin Cancer
- The Cerebral Vasculature System in Disease: Atherosclerosis
- DNA Repair Mechanisms
- Beyond Pigment: the Melanocortin 1 Receptor (MC1R) in DNA Repair
- Photoprotection and the significance of Minimal Erythema Dose (MED) testing
- Developing Novel Treatments for Arterial Ischaemic Stroke Patients